Biology and Metabolism of Muscle Tissue
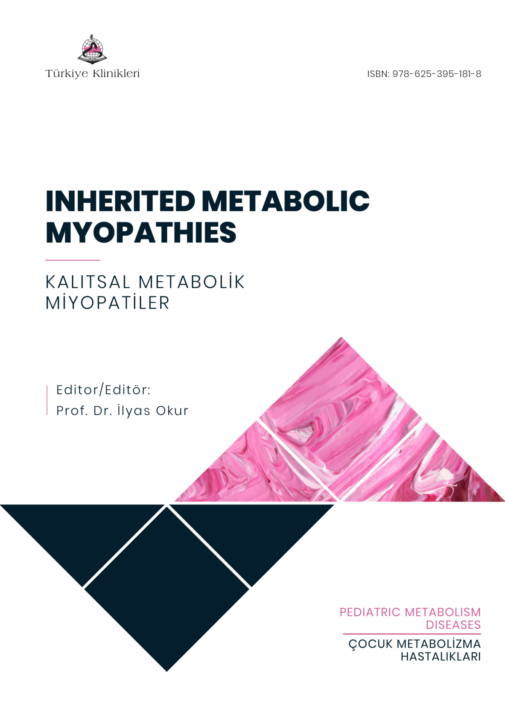
Atiye Seda YAR SAĞLAMa , Duygu Deniz USTA SALİMİa,b
aGazi University Faculty of Medicine, Department of Medical Biology, Ankara, Türkiye
bİstanbul Medeniyet University Faculty of Medicine, Department of Medical Biology, İstanbul, Türkiye
ABSTRACT
Muscle tissue, a fundamental component of the human body, plays a multifaceted role in maintaining physiological homeostasis and enabling essential functions. It enables a wide range of activities, from the simplest actions like walking to the execution of fine motor skills. Integral to the respiratory system, it enables the essential exchange of oxygen and removal of carbon dioxide through respiration. Specialized form as cardiac muscle, ensuring the circulation of blood throughout the body, thereby guaranteeing the delivery of oxygen and nutrients to all bodily tissues and organs. It significantly contributes to the digestive process by coordinating peristaltic contractions. Furthermore, it plays a pivotal role in the body’s thermoregulatory mechanisms, generating heat during contractions. During muscle contractions, glucose, glycogen stored in muscles, and fatty acids are utilized, making them integral components of muscle cell metabolism. The interconnected biology and metabolism of muscle tissue are indispensable for sustaining human health and function.
Keywords: Muscle tissue; skeletal muscle; cardiac muscle; smooth muscle; biology and metabolism of muscle tissue
Referanslar
- Alberts B, Johnson A, Lewis J, Morgan D, Roberts MK, Walter P. Molecular Biology of the Cell. 6th ed. 2014. p.889-962.
- Cooper GM, Hausman RE. The Cell: A Molecular Approach. 8th ed. 2019. p.453-500.
- Noyan A. Yaşamda ve Hekimlikte Fizyoloji. 17. Baskı. Ankara: Meteksan A.Ş.; 2008.
- Westerblad H, Bruton JD, Katz A. Skeletal muscle: energy metabolism, fiber types, fatigue and adaptability. Exp Cell Res. 2010;316(18):3093-9. [Crossref] [PubMed]
- Mukund K, Subramaniam S. Skeletal muscle: A review of molecular structure and function, in health and disease. Wiley Interdiscip Rev Syst Biol Med. 2020;12(1):e1462. [Crossref] [PubMed] [PMC]
- Sobue K, Hayashi K, Nishida W. Molecular mechanism of phenotypic modulation of smooth muscle cells. Horm Res. 1998;50(2):15-24. [Crossref] [PubMed]
- Campbell N, Reece J. Campbell Biology. 6th ed. 2006. p.1075-87.
- Koubassova NA, Tsaturyan AK. Molecular Dynamics Assessment of Mechanical Properties of the Thin Filaments in Cardiac Muscle. Int J Mol Sci. 2023;24(5):4792. [Crossref] [PubMed] [PMC]
- Yamada Y, Namba K, Fujii T. Cardiac muscle thin filament structures reveal calcium regulatory mechanism. Nat Commun. 2020;11(1):153. [Crossref] [PubMed] [PMC]
- Brunello E, Fusi L, Ghisleni A, Park-Holohan SJ, Ovejero JG, et al. Myosin filament-based regulation of the dynamics of contraction in heart muscle. PNAS. 2020;117(14):8177-86. [Crossref] [PubMed] [PMC]
- Hall JE. Textbook of Medical Physiology. 13th ed. 2016. p.75-88.
- McNamara JW, Singh RR, Sadayappan S. Cardiac myosin binding protein-C phosphorylation regulates the super-relaxed state of myosin. PNAS. 2019;116(24):11731-36. [Crossref] [PubMed] [PMC]
- Herrera AM, McParland BE, Bienkowska A, Tait R, Paré PD, Seow CY. 'Sarcomeres' of smooth muscle: functional characteristics and ultrastructural evidence. J Cell Sci. 2005;118(11):2381-92. [Crossref] [PubMed]
- Billups D, Billups B, Challiss RA, Nahorski SR. Modulation of Gq-protein-coupled inositol trisphosphate and Ca2+ signaling by the membrane potential. J Neurosci. 2006;26(39):9983-95. [Crossref] [PubMed] [PMC]
- He WQ, Peng YJ, Zhang WC, Lv N, Tang J, Chen C, et al. Myosin light chain kinase is central to smooth muscle contraction and required for gastrointestinal motility in mice. Gastroenterology. 2008;135(2):610-20. [Crossref] [PubMed] [PMC]
- Matchkov VV, Kudryavtseva O, Aalkjaer C. Intracellular Ca²⁺ signalling and phenotype of vascular smooth muscle cells. Basic Clin Pharmacol Toxicol. 2012;110(1):42-8. [Crossref] [PubMed]
- Tang DD. Critical role of actin-associated proteins in smooth muscle contraction, cell proliferation, airway hyperresponsiveness and airway remodeling. Respir Res. 2015;16:134. [Crossref] [PubMed] [PMC]
- Tobacman LS. Troponin Revealed: Uncovering the Structure of the Thin Filament On-Off Switch in Striated Muscle. Biophys J. 2021;120(1):1-9. [Crossref] [PubMed] [PMC]
- Gordon AM, Homsher E, Regnier M. Regulation of contraction in striated muscle. Physiol Rev. 2000;80(2):853-924. [Crossref] [PubMed]
- Vinogradova MV, Stone DB, Malanina GG, Karatzaferi C, Cooke R, Mendelson RA, et al. Ca(2+)-regulated structural changes in troponin. Proc Natl Acad Sci U S A. 2005;102(14):5038-43. [Crossref] [PubMed] [PMC]
- Berne RM, Levy MN, Koeppen BM, Stanton BA. Fizyoloji. 5. Baskı.2008. p.223-62.
- Marieb EN, Hoehn K. Human Anatomy & Physiology. 10th ed. 2015. p.298-340.
- Tortora GJ, Derrickson B. Principles of Anatomy &Physiology. 15th ed. 2017. p.135-7, 293-396.
- Powers JD, Malingen SA, Regnier M, Daniel TL. The Sliding Filament Theory Since Andrew Huxley: Multiscale and Multidisciplinary Muscle Research. Annu Rev Biophys. 2021;50:373-400. [Crossref] [PubMed]
- Marcucci L. Muscle Mechanics and Thick Filament Activation: An Emerging Two-Way Interaction for the Vertebrate Striated Muscle Fine Regulation. Int J Mol Sci. 2023;24(7):6265. [Crossref] [PubMed] [PMC]
- Brunello E, Caremani M, Melli L, Linari M, Fernandez-Martinez M, et al. The contributions of filaments and cross-bridges to sarcomere compliance in skeletal muscle. J Physiol. 2014;592(17):3881-99. [Crossref] [PubMed] [PMC]
- Shi J, Yang Y, Cheng A, Xu G, He F. Metabolism of vascular smooth muscle cells in vascular diseases. Am J Physiol Heart Circ Physiol. 2020;319(3):613-31. [Crossref] [PubMed]
- Hall JL, Chatham JC, Eldar-Finkelman H, Gibbons GH. Upregulation of glucose metabolism during intimal lesion formation is coupled to the inhibition of vascular smooth muscle cell apoptosis. Role of GSK3beta. Diabetes. 2001;50(5):1171-9. [Crossref] [PubMed]
- Paul RJ, Bauer M, Pease W. Vascular smooth muscle: aerobic glycolysis linked to sodium and potassium transport processes. Science. 1979;206(4425):1414-6. [Crossref] [PubMed]
- Paul RJ, Krisanda JM, Lynch RM. Vascular smooth muscle energetics. J Cardiovasc Pharmacol. 1984;6(2):S320-7. [Crossref] [PubMed]
- Paul RJ. Functional compartmentalization of oxidative and glycolytic metabolism in vascular smooth muscle. Am J Physiol. 1983;244(5):C399-409. [Crossref] [PubMed]
- Scopes RK. Studies with a reconstituted muscle glycolytic system. The rate and extent of creatine phosphorylation by anaerobic glycolysis. Biochem J. 1973;134(1):197-208. [Crossref] [PubMed] [PMC]
- Ishida Y, Riesinger I, Wallimann T, Paul RJ. Compartmentation of ATP synthesis and utilization in smooth muscle: roles of aerobic glycolysis and creatine kinase. Mol Cell Biochem. 1994;133-134:39-50. [Crossref] [PubMed]
- Guimarães-Ferreira L. Role of the phosphocreatine system on energetic homeostasis in skeletal and cardiac muscles. Einstein (Sao Paulo). 2014;12(1):126-31. [Crossref] [PubMed] [PMC]
- Bessman SP, Geiger PJ. Transport of energy in muscle; the phosphorylcreatine shuttle. Science. 1981;211(4481):448-52. [Crossref] [PubMed]