Climate Change and Epigenetics
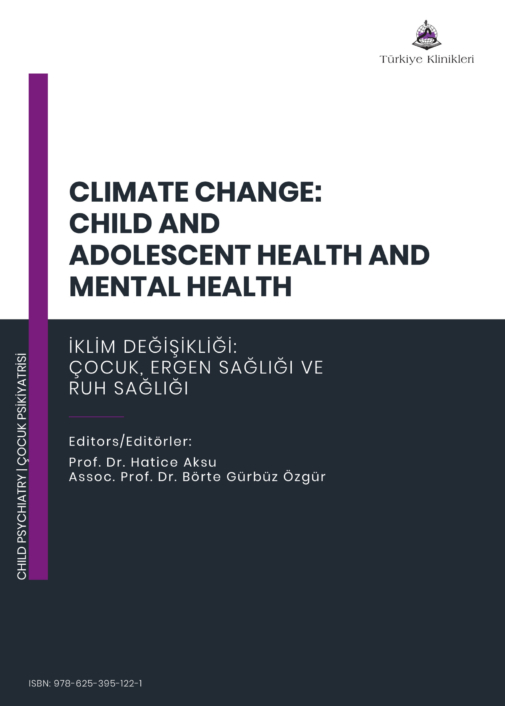
Tarık DÜZENLİa, Ferda Emriye PERÇİNb
aGazi University Faculty of Medicine, Department of Medical Genetics, Ankara, Türkiye
bGENTAN Genetic Diseases Evaluation Center, İzmir, Türkiye
ABSTRACT
Climate change is a continuous global phenomenon that has significant implications on various aspects of human life. It has both direct and indirect effects on human health. The effects of climate change on human health are not only limited to the exposed generation, but also have the potential to affect future generations through epigenetic inheritance. Epigenetics refers to heritable changes that regulate gene expression and phenotype without altering the primary DNA sequence. Epigenetic modifications play a crucial role in the regulation of the developmental process, tuning the physiological gene dosage required for the maintenance of vital functions, and adaptation of organisms to changes in environmental conditions. However, it has also been shown that epigenetic inheritance causes the transmission of some pathological conditions, which can also be defined as maladaptation, to future generations. Recent studies and scientific evidence prove that climate change has effects on human physiology through epigenetic changes. Therefore, the effects of the external environment on genes may play a role in disease etiopathogenesis in humans and some of these effects may be heritable. In support of this, there is growing evidence that disruption of gene expression patterns governed by epigenetics can lead to autoimmune diseases, metabolic and psychiatric disorders and cancers. Further delineation of epigenetic mechanisms that underlie disorders may also enhance our understanding of the pathogenesis of novel treatments that target these specific mechanisms.
Keywords: Climate change; epigenomics; epigenesis, genetic
Citation
Referanslar
- Intergovernmental Panel on Climate, C. Global Warming of 1.5°C: IPCC Special Report on Impacts of Global Warming of 1.5°C above Pre-industrial Levels in Context of Strengthening Response to Climate Change, Sustainable Development, and Efforts to Eradicate Poverty (Cambridge University Press). 2022.
- Smith ZD, Meissner A. DNA methylation: roles in mammalian development. Nat Rev Genet. 2013;14(3):204-20. [Crossref] [PubMed]
- Unal E, Arbel-Eden A, Sattler U, Shroff R, Lichten M, Haber JE, et al. DNA damage response pathway uses histone modification to assemble a double-strand break-specific cohesin domain. Mol Cell. 2004;16(6):991-1002. [Crossref] [PubMed]
- Horvath S, Raj K. DNA methylation-based biomarkers and the epigenetic clock theory of ageing. Nat Rev Genet. 2018;19(6):371-84. [Crossref] [PubMed]
- Kanherkar RR, Bhatia-Dey N, Csoka AB. Epigenetics across the human lifespan. Front Cell Dev Biol. 2014;2:49. [Crossref] [PubMed] [PMC]
- Waddington CH. The strategy of the genes; a discussion of some aspects of theoretical biology (Allen & Unwin). 1957.
- Waddington CH. Canalization Of Development And The Inheritance Of Acquired Characters. Nature. 1942;150:563-5. [Crossref]
- Waddington CH. Genetic Assimilation of an Acquired Character. JSTOR. 1953;7:118-26. [Crossref]
- Chen T, Li E. Structure and function of eukaryotic DNA methyltransferases. Curr Top Dev Biol. 2004;60:55-89. [Crossref] [PubMed]
- Saxonov S, Berg P, Brutlag DL. A genome-wide analysis of CpG dinucleotides in the human genome distinguishes two distinct classes of promoters. Proc Natl Acad Sci U S A. 2006;103(5):1412-7. [Crossref] [PubMed] [PMC]
- Al Aboud NM, Tupper C, Jialal I. Genetics, Epigenetic Mechanism. 2023 Aug 14. In: StatPearls [Internet]. Treasure Island (FL): StatPearls Publishing; 2023.
- Yang X, Han H, De Carvalho DD, Lay FD, Jones PA, Liang G. Gene body methylation can alter gene expression and is a therapeutic target in cancer. Cancer Cell. 2014;26(4):577-90. [Crossref] [PubMed] [PMC]
- Zhao H, Chen T. Tet family of 5-methylcytosine dioxygenases in mammalian development. J Hum Genet. 2013;58(7):421-7. [Crossref] [PubMed] [PMC]
- Rossetto D, Avvakumov N, Côté J. Histone phosphorylation: a chromatin modification involved in diverse nuclear events. Epigenetics. 2012;7(10):1098-108. [Crossref] [PubMed] [PMC]
- Bannister AJ, Kouzarides T. Regulation of chromatin by histone modifications. Cell Res. 2011;21(3):381-95. [Crossref] [PubMed] [PMC]
- Frías-Lasserre D, Villagra CA. The Importance of ncRNAs as Epigenetic Mechanisms in Phenotypic Variation and Organic Evolution. Front Microbiol. 2017;8:2483. [Crossref] [PubMed] [PMC]
- Wei JW, Huang K, Yang C, Kang CS. Non-coding RNAs as regulators in epigenetics (Review). Oncol Rep. 2017;37(1):3-9. [Crossref] [PubMed]
- Chhabra R. miRNA and methylation: a multifaceted liaison. Chembiochem. 2015;16(2):195-203. [Crossref] [PubMed]
- Braconi C, Huang N, Patel T. MicroRNA-dependent regulation of DNA methyltransferase-1 and tumor suppressor gene expression by interleukin-6 in human malignant cholangiocytes. Hepatology. 2010;51(3):881-90. [Crossref] [PubMed] [PMC]
- Fabbri M, Garzon R, Cimmino A, Liu Z, Zanesi N, Callegari E, et al. MicroRNA-29 family reverts aberrant methylation in lung cancer by targeting DNA methyltransferases 3A and 3B. Proc Natl Acad Sci U S A. 2007;104(40):15805-10. [Crossref] [PubMed] [PMC]
- D'Urso A, Brickner JH. Mechanisms of epigenetic memory. Trends Genet. 2014;30(6):230-6. [Crossref] [PubMed] [PMC]
- Brickner DG, Cajigas I, Fondufe-Mittendorf Y, Ahmed S, Lee PC, Widom J, et al. H2A.Z-mediated localization of genes at the nuclear periphery confers epigenetic memory of previous transcriptional state. PLoS Biol. 2007;5(4):e81. [Crossref] [PubMed] [PMC]
- Youngson NA, Whitelaw E. Transgenerational epigenetic effects. Annu Rev Genomics Hum Genet. 2008;9:233-57. [Crossref] [PubMed]
- Conine CC, Rando OJ. Soma-to-germline RNA communication. Nat Rev Genet. 2022;23(2):73-88. [Crossref] [PubMed]
- Sharma U, Sun F, Conine CC, Reichholf B, Kukreja S, Herzog VA, et al. Small RNAs Are Trafficked from the Epididymis to Developing Mammalian Sperm. Dev Cell. 2018;46(4):481-94.e6. [Crossref] [PubMed] [PMC]
- Sharma A. Transgenerational epigenetic inheritance: focus on soma to germline information transfer. Prog Biophys Mol Biol. 2013;113(3):439-46. [Crossref] [PubMed]
- Fitz-James MH, Cavalli G. Molecular mechanisms of transgenerational epigenetic inheritance. Nat Rev Genet. 2022;23(6):325-31. [Crossref] [PubMed]
- Heard E, Martienssen RA. Transgenerational epigenetic inheritance: myths and mechanisms. Cell. 2014;157(1):95-109. [Crossref] [PubMed] [PMC]
- Skvortsova K, Iovino N, Bogdanović O. Functions and mechanisms of epigenetic inheritance in animals. Nat Rev Mol Cell Biol. 2018;19(12):774-90. [Crossref] [PubMed]
- Francis D, Diorio J, Liu D, Meaney MJ. Nongenomic transmission across generations of maternal behavior and stress responses in the rat. Science. 1999;286(5442):1155-8. [Crossref] [PubMed]
- Weaver IC, Cervoni N, Champagne FA, D'Alessio AC, Sharma S, Seckl JR, et al. Epigenetic programming by maternal behavior. Nat Neurosci. 2004;7(8):847-54. [Crossref] [PubMed]
- Weaver IC, Meaney MJ, Szyf M. Maternal care effects on the hippocampal transcriptome and anxiety-mediated behaviors in the offspring that are reversible in adulthood. Proc Natl Acad Sci U S A. 2006;103(9):3480-5. [Crossref] [PubMed] [PMC]
- Soga T, Teo CH, Parhar I. Genetic and Epigenetic Consequence of Early-Life Social Stress on Depression: Role of Serotonin-Associated Genes. Front Genet. 2021;11:601868. [Crossref] [PubMed] [PMC]
- Yehuda R, Lehrner A. Intergenerational transmission of trauma effects: putative role of epigenetic mechanisms. World Psychiatry. 2018;17(3):243-57. [Crossref] [PubMed] [PMC]
- Yehuda R, Daskalakis NP, Lehrner A, Desarnaud F, Bader HN, Makotkine I, et al. Influences of maternal and paternal PTSD on epigenetic regulation of the glucocorticoid receptor gene in Holocaust survivor offspring. Am J Psychiatry. 2014;171(8):872-80. [Crossref] [PubMed] [PMC]
- Yehuda R, Daskalakis NP, Bierer LM, Bader HN, Klengel T, Holsboer F, et al. Holocaust Exposure Induced Intergenerational Effects on FKBP5 Methylation. Biol Psychiatry. 2016;80(5):372-80. [Crossref] [PubMed]
- Dias BG, Ressler KJ. Parental olfactory experience influences behavior and neural structure in subsequent generations. Nat Neurosci. 2014;17(1):89-96. [Crossref] [PubMed] [PMC]
- Khogeer AA, AboMansour IS, Mohammed DA. The Role of Genetics, Epigenetics, and the Environment in ASD: A Mini Review. Epigenomes. 2022;6(2):15. [Crossref] [PubMed] [PMC]
- Zhu Y, Mordaunt CE, Yasui DH, Marathe R, Coulson RL, Dunaway KW, et al Placental DNA methylation levels at CYP2E1 and IRS2 are associated with child outcome in a prospective autism study. Hum Mol Genet. 2019;28(16):2659-74. [Crossref] [PubMed] [PMC]
- Schraufnagel DE, Balmes JR, Cowl CT, De Matteis S, Jung SH, Mortimer K, et al. Air Pollution and Noncommunicable Diseases: A Review by the Forum of International Respiratory Societies' Environmental Committee, Part 2: Air Pollution and Organ Systems. Chest. 2019;155(2):417-26. [Crossref] [PubMed] [PMC]
- Kohli RM, Zhang Y. TET enzymes, TDG and the dynamics of DNA demethylation. Nature. 2013;502(7472):472-9. [Crossref] [PubMed] [PMC]
- Leclercq B, Platel A, Antherieu S, Alleman LY, Hardy EM, Perdrix E, et al. Genetic and epigenetic alterations in normal and sensitive COPD-diseased human bronchial epithelial cells repeatedly exposed to air pollution-derived PM2.5. Environ Pollut. 2017;230:163-77. [Crossref] [PubMed]
- Liang Y, Zeng J, Luo B, Li W, He Y, Zhao W, et al. TET2 promotes IL-1β expression in J774.1 cell through TLR4/MAPK signaling pathway with demethylation of TAB2 promoter. Mol Immunol. 2020;126:136-42. [Crossref] [PubMed]
- Chen R, Meng X, Zhao A, Wang C, Yang C, Li H, et al. DNA hypomethylation and its mediation in the effects of fine particulate air pollution on cardiovascular biomarkers: A randomized crossover trial. Environ Int. 2016;94:614-9. [Crossref] [PubMed]
- Cai J, Zhao Y, Liu P, Xia B, Zhu Q, Wang X, et al. Exposure to particulate air pollution during early pregnancy is associated with placental DNA methylation. Sci Total Environ. 2017;607-608:1103-8. [Crossref] [PubMed]
- Zhong J, Karlsson O, Wang G, Li J, Guo Y, Lin X, et al. B vitamins attenuate the epigenetic effects of ambient fine particles in a pilot human intervention trial. Proc Natl Acad Sci U S A. 2017;114(13):3503-8. [Crossref] [PubMed] [PMC]
- Tsai MH, Chi MC, Hsu JF, Lee IT, Lin KM, Fang ML, et al. Urban Particulate Matter Enhances ROS/IL-6/COX-II Production by Inhibiting MicroRNA-137 in Synovial Fibroblast of Rheumatoid Arthritis. Cells. 2020;9(6):1378. [Crossref] [PubMed] [PMC]
- Espin-Perez A, Krauskopf J, Chadeau-Hyam M, van Veldhoven K, Chung F, Cullinan P, et al. Short-term transcriptome and microRNAs responses to exposure to different air pollutants in two population studies. Environ Pollut. 2018;242:182-90. [Crossref] [PubMed]
- Tiihonen J, Halonen P, Tiihonen L, Kautiainen H, Storvik M, Callaway J. The Association of Ambient Temperature and Violent Crime. Sci Rep. 2017;7(1):6543. [Crossref] [PubMed] [PMC]
- Dey T, Zanobetti A, Linnman C. The risk of being bitten by a dog is higher on hot, sunny, and smoggy days. Sci Rep. 2023;13:8749. [Crossref]
- Lim YH, Han C, Bae S, Hong YC. Modulation of blood pressure in response to low ambient temperature: The role of DNA methylation of zinc finger genes. Environ Res. 2017;153:106-11. [Crossref] [PubMed]
- Våge J, Bønsdorff TB, Arnet E, Tverdal A, Lingaas F. Differential gene expression in brain tissues of aggressive and non-aggressive dogs. BMC Vet Res. 2010;6:34. [Crossref] [PubMed] [PMC]
- Richardson B. The interaction between environmental triggers and epigenetics in autoimmunity. Clin Immunol. 2018;192:1-5. [Crossref] [PubMed] [PMC]
- Cao-Lei L, Massart R, Suderman MJ, Machnes Z, Elgbeili G, Laplante DP, et al. DNA methylation signatures triggered by prenatal maternal stress exposure to a natural disaster: Project Ice Storm. PLoS One. 2014;9(9):e107653. [Crossref] [PubMed] [PMC]
- Lumey LH, Stein AD, Susser E. Prenatal famine and adult health. Annu Rev Public Health. 2011;32:237-62. [Crossref] [PubMed] [PMC]
- Tobi EW, Slieker RC, Luijk R, Dekkers KF, Stein AD, Xu KM; Biobank-based Integrative Omics Studies Consortium; Slagboom PE, van Zwet EW, Lumey LH, Heijmans BT. DNA methylation as a mediator of the association between prenatal adversity and risk factors for metabolic disease in adulthood. Sci Adv. 2018;4(1):eaao4364. [Crossref] [PubMed] [PMC]
- Wang Z, Song J, Li C, Li Y, Shen L, Dong B, et al. DNA methylation of the INSR gene as a mediator of the association between prenatal exposure to famine and adulthood waist circumference. Sci Rep. 2020;10(1):12212. [Crossref] [PubMed] [PMC]
- Jiang W, Han T, Duan W, Dong Q, Hou W, Wu H, et al. Prenatal famine exposure and estimated glomerular filtration rate across consecutive generations: association and epigenetic mediation in a population-based cohort study in Suihua China. Aging (Albany NY). 2020;12(12):12206-21. [Crossref] [PubMed] [PMC]
- Mora C, Spirandelli D, Franklin EC, Lynham J, Kantar MB, Miles W, et al. Broad threat to humanity from cumulative climate hazards intensified by greenhouse gas emissions. Nature Climate Change. 2018;8:1062-71. [Crossref]
- Straight B, Qiao X, Ngo D, Hilton CE, Olungah CO, Naugle A, et al. Epigenetic mechanisms underlying the association between maternal climate stress and child growth: characterizing severe drought and its impact on a Kenyan community engaging in a climate change-sensitive livelihood. Epigenetics. 2022;17(13):2421-33. [Crossref] [PubMed] [PMC]